- Why Focus on the Future of Food?
- Weed Management
- How Best to Breed and Grow Healthy Plants
- “New Age” Industrial Food
- Nutrition — Toward Healthier Fats
Why Focus on the Future of Food?
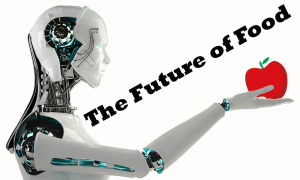
Major crop yields have plateaued in most countries. The nutrient content of conventionally grown food has declined markedly, with no end in sight because of climate change.
A growing global population needs to be fed while balancing the need for environmental protection and habitat conservation.
Plus, widely used weed management tools are failing us. Weed scientists are openly asking what comes next, after the last herbicide still standing becomes uneconomical because of too many resistant weeds.
Challenges abound, and many are looking to technology to turn the tide.
Science is making real progress and technology marches on, but in what direction, for what purpose, and serving whose needs?
Drones are diagnosing yield-threatening pest infestations, drought conditions, and where too much or not enough fertilizer has been applied. Others are delivering sandwiches on 5th Avenue.
Companies developing artificial intelligence-based technology see agriculture and the food industry as huge, future markets. Already, robots are flipping burgers in a few fast food restaurants.
Glossy videos and glowing media stories tell of technological advances that are, for sure this time, going to solve agriculture’s biggest problems, and pave the way towards a brighter tomorrow.
One example — the biotech industry is pushing hard to bring a new generation of genetically-engineered oilseed crops to market that produce oils that do not require partial hydrogenation, and hence last longer in deep-fat fryers. But soybean oil hydrogenation is an industrial process that produces trans fats linked to heart disease and cancer. Conventional soybean breeders are in the hunt as well.
Several companies are developing biopesticides that are permitted for use in organic farming because they come from natural sources, and do not contain any synthetic chemical ingredients. They work by altering microbial and pest interactions, both above and below ground. They don’t kill anything directly, they just tip the competitive balance toward beneficial critters at the expense of harmful ones.
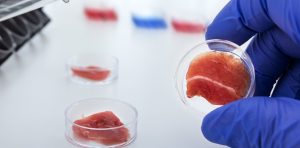
A growing diversity of entrepreneurs think they can come up with better, high-tech solutions to what ails agriculture. And some are growing artificial food, especially meat, using genetically altered microorganisms in large metal vats. And how about fresh cucumbers or strawberries from soil-less hydroponic systems on rooftops in Manhattan?
The Future of Food is inseparable from the future of people, animals, and the planet. All living things, and the systems supporting life on earth, will be impacted in a myriad of ways by which of these new-age technologies prove ready for prime time and are widely adopted, and which fall by the wayside.
Much of the world’s farmland is in developing countries, as are most of the chronically malnourished. How will high-tech agriculture influence farmers in regions that are still making the shift to “modern” farming methods? Ripple affects spanning culture, social structures, power relationships, health, the environment, and economics will be complex, sometimes disruptive, but also hopefully healing. While the focus of the Future of Food is the U.S. food system, the impacts of new-age technology in developing countries will also be explored from time to time.
We all hope that just those technologies that are truly safe and sustainable, humane, and profitable at the farm level, will make it into widespread use. We can also hope for world peace, a cure for diabetes, and electricity too cheap to meter.
The Future of Food is an enormously complex, dynamic topic. We will address it in bite-sized chunks, starting with three key pressing challenges: 1) Dealing with Weeds, 2) How Best to Breed and Grow Plants, and 3) How to Improve the Nutritional Quality of Fat in the American Diet. Look for more content in the future — we’ll alert you when new items post if you sign up for our contact list.
Dealing with Weeds
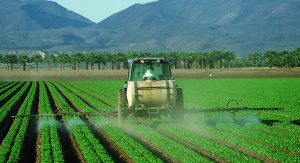
Herbicides have been the go-to tools for weed management on most commercial farms for decades.
Heavy use of the triazines, glyphosate, dicamba, and 2,4-D has resulted in a bevy of weeds developing resistance to one or more herbicides.
As a result, many farmers are trapped on a herbicide treadmill that is clearly not sustainable from the perspectives of efficacy, cost to farmers, or adverse impacts on human health and the environment.
Next-Gen Herbicides
In a generally failing effort to keep ahead of weeds now resistant to multiple herbicides, the pesticide-biotech-seed industry is investing heavily in a new suite of next-gen herbicides designed to go along with next-gen GMO crops bred to be resistant to multiple herbicides.
Farmers have incrementally more narrow options, since the now-four major players in the industry are the ones who decide which resistant genes to move into a given crop variety, and over 90% of the corn, soybean, cotton, alfalfa, and sugar beet seed sold in the U.S. is expressing one, or more likely, several GE traits (some up to six).
When ordering seed, farmers cannot ask their seed dealer to “hold” a GE trait that the farmer does not want to pay for, because she or he does not need the trait, and/or does not want to flirt with its unintended consequences (for more, see “Section 3B. Next Gen GE-HR Crops” on the “Managing Weeds for Healthy Kids” website.
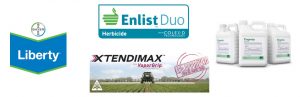
Space-Age Tillage Tools
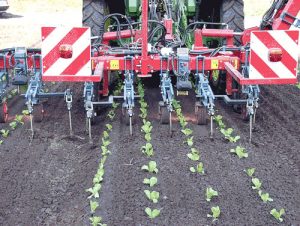
Over the arc of history, most weeds have been controlled through crop rotation, human weeding, mechanical tillage tools, or just tolerated. Herbicides are a relatively recent technology.
“Peak” efficacy and dependence on herbicides in managing weeds in corn, soybeans, and cotton has almost certainly already occurred. More and more farmers have realized that relying too heavily on herbicides — and dropping other control measures — is a flawed strategy that will assure the emergence and spread of resistant weeds.H herbicide treadmill is great for the gross sales and profit margins of the companies selling GMO, herbicide-resistant seed and the herbicides that go along with them. But not so good for farmers, rural communities and neighbors, public health, and the environment.
It is encouraging that the simple phrase “many little hammers” is making a comeback in characterizing proven alternatives to excessive reliance on herbicides.
Hi-tech tillage tools are already among the new-age “hammers” that some farmers are using. These new machines use sensors, GPS, and other technologies to do precisely and quickly what any of us on the working end of a hoe can do in the garden. But how such machines will fit into the future of weed management remains unclear, subject to many “what ifs.”
Artificial Intelligence
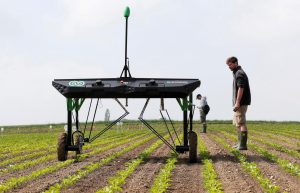
Here’s where the weed-management toolbox starts to enter the Twilight Zone. Are you ready for weed killing robots?
Artificial intelligence (A.I.) is exploding right now, and the agricultural sector is getting its share of attention.
While innovations in machinery have been a critical part of the development of industrial ag since the introduction of the grain threshing machine and the cotton gin, A.I. has only recently become an option.
Several small companies are racing to develop market-ready, A.I.-based weed management prototypes, and big ag is starting to sit up and take notice. “Robots, Coming Soon to a Field Near You” includes videos of several prototype, robot weeders.
Other resources:
- Is a wave of robot farmers going to solve our farm labor problems?, CNBC, 2018
- Ag robots are a hot topic in Australia-
- “These Robots Can Redefine The Future Of Farming, Say Sydney Researchers,” from June 2018
- Australian organic farmers will get to try out robot weeders in 2018, courtesy of a government grant
- Plus, robots are a big player in food processing and production, as the Wall Street Journal reports (2018)
Breeding and Growing Healthy Plants
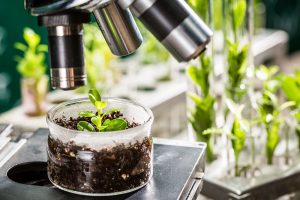
A wise person once said, in addressing how to solve production-based problems on the farm, “Don’t use a genetic solution to solve a management problem.” (The wise person passing on this simple and important insight to me, some 25 years ago, was Dr. Anne Clark).
Why is this statement so important? Because it recognizes that the use of plant breeding of all sorts should be focused on those problems that farmers cannot solve on their own via changes in their management systems.
It also recognizes that in plant modification, there are no free lunches. Any change in the genetics of a crop or animal will have a myriad of impacts, some desired and positive, others neutral, some negative, and others unknowable.
Plus, even with today’s powerful new tools, plant breeders can only focus on changing a discrete subset of traits at any one time, when dozens of traits are important.
Almost certainly, the most consequential example in the history of mankind of the impact of choices made by plant breeders will be the era of first-generation GE crops — circa 1990 through 20??.
Ag historians of the future will eventually piece together the impacts in the U.S. over the last 40-50 years, as most investment and leadership in plant breeding was transferred from public institutions to the private sector, and targeted mostly on the development of herbicide-resistant and Bt-transgenic corn, soybeans, and cotton varieties. The judgement of history is not likely to align with the contemporary PR from the biotech industry and its cheerleaders.
Conventional Breeding
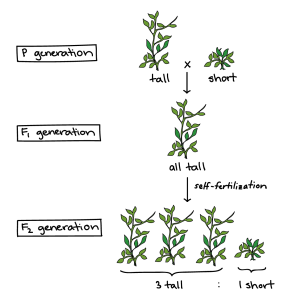
“Conventional” breeding refers to the way plant breeders have selected for higher yielding, hardier, and hopefully healthier plants and animals since Mendel first dabbled with pea plants in a Moravian abbey garden.
This is still by far the most important way both farmers and scientists develop “improved” varieties, and also the major way they inadvertently produce crops and animals that are less nutritious, more prone to certain pests and diseases, and/or more dependent on “crutches” from off the farm.
Conventional breeding involves the conscious exploitation of genetic variation within the genome of a crop, or species of animal, and the selection of plants and animals that appear superior, because they are better suited to thrive within their environment, and/or more fully meet the needs and hopes of farmers.
Conventional breeding depends on genetic variation that exists within sexually compatible populations of plants and animals, coupled with the annual “supply” of mutations that happen in the real world, and become part of the genetic diversity that breeders can draw upon.
Some tools used by conventional breeders artificially increase the rate of mutations within a crop genome, to increase the “supply” of possibly helpful mutations. Other innovations over the last few decades have empowered conventional breeders with precise new tools to track the linkages between a desired — or undesirable — trait and the gene or genes giving rise to it. These new techniques and technologies comprise the toolbox of what is called “marker-assisted” breeding.
For at least the next several decades, ongoing innovation in conventional breeding technology and methods will continue to drive the future of food more so than any other breeding technology.
Biotech
We cover the development of early GMO products like Roundup Ready and Bt crops extensively in our Ag Biotech section. These “innovations” were adopted widely and quickly. By the end of the first decade of Roundup Ready crops, glyphosate had become the #1 pesticide measured by volume applied (it was #7 in 1995, one year before glyphosate-resistant crops hit the market). In 2006, over 90% of soybean acres nationwide were sprayed with glyphosate.
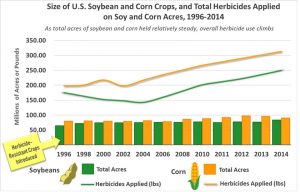
This surge in herbicide use has come with many unintended consequences. The rise of resistant weeds, and a herbicide treadmill moving into hyper-drive are the most serious.
Despite wishful thinking and funny-math PR from the pesticide-biotech-seed industry, farmers are spraying more herbicides than ever. As the search continues for new weed management systems — and for new and better “little hammers” — who will decide where public and private sector R&D investments are deployed? Who will benefit, and who will bear the costs and risks?
A transgene is a gene that is transferred from one organism into another by scientists using the tools of genetic engineering, thus producing a GE or GMO organism, usually a plant, animal, or bacterium. Transgenes are used to transfer pieces of DNA with known, desired functions into an organism that lacks those functions.
Getting the transgene into the DNA of the target organism poses a first set of technical challenges. Assuring that the new gene transfers to a location where it will be properly expressed, but not excessively, and without disrupting existing genes, is another highly complex challenge.
CRISPR and Other Gene Editing Tools
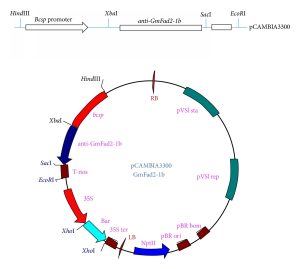
CRISPR gene editing is a tool for making changes in the genetic code and expression of genes that already exist within a plant or animal genome. Like conventional breeding, gene editing tools work with the genetic “hand” nature has dealt.
This technology silences or changes the expression of genes that already reside in a crop or animal genome, or alters their sequence to be identical to similar genes that may be found in a different members of the species.
They can also be used to insert transgenes from other species into plants, and do it in designated places in the genome, unlike previous transgenesis. In humans, it also has been used to repair genetic defects.
One area of uncertainty — will some applications of “gene editing” become functionally indistinguishable from contemporary biotech?
Indeed, it seems likely that gene editing will be used in combination with transgene-driven genetic transformations in major food crops. The combination of gene editing and transgene alteration of plant genomes is sure to trigger a next-gen set of unintended consequences. They will likely be largely ignored for the same reasons the unintended consequences of first-generation GE transformations were ignored.
Despite the reassuring rhetoric from the biotech industry and its advocates, scientists still do not understand the unintended consequences of “stacking” multiple GE traits into a single GMO plant variety. Now, they will have to sort out how gene editing interventions impact both the expression of existing genes in a plant genome, and the novel genes moved into the genome via transgenes.
One prediction is a no brainer — expect surprises, not all good.
In 2017, we reviewed a report by GM Watch that summarized many of the concerns about gene editing technologies, and it is a good place to start to see what the fuss is all about. With the benefit of “gene editing 101” in the GM Watch report, gain further insights elsewhere on Hygeia:
- “Mathematical Model Predicts Errors in Crispr-Cas9 DNA Targeting,” 2018 Hot Science post
- 2016 post on Monsanto’s first commercial license for CRISPR technology, “Important Developments on the CRISPR Front”
- Plant breeding for more productive organic crops, peer-reviewed paper published in 2015 in Trends in Plant Science
“New Age” Industrial Food
The industrialization of the U.S. food and agricultural system has been going on for a half-century. Its hallmarks include:
- Larger, more specialized farms that are heavily dependent on off-farm inputs to “feed” crops and protect against pest and animal disease loses;
- The trend toward bigger tractors, bigger combines, wider planters and spray rigs, higher volume manure wagons, and many other sorts of always-bigger mechanical tools;
- Confined animal feeding operations (CAFOs) that house thousands of animals in relatively small spaces, and strive, first and foremost, to reduce the hours of labor needed per unit of eggs, chicken, beef, pork, or milk produced;
- Specialized food manufacturing plants that can process a large volume of a specific, raw, agricultural commodity, along with secondary ingredients, into packaged, ready-to-sell brand-name products, quickly; and
- Sophisticated, tightly integrated supply chains through which food harvested on a farm winds up on a consumer’s plate, or in a meal at a restaurant.
We are clearly entering a new phase in the industrialization of the food system. Three powerful new technologies will make a mark on the future of food — sensor technology and “big” data, growing food in vats using genetically engineered microorganisms, and growing plants that never come in contact with the soil.
Our initial focus will be on food that is grown in a substantially different way than in the past. One example — Strawberries, tomatoes, eggplant and cucumbers grown in hydroponic systems in which roots never touch soil are one example. Such systems are not just soil-less, some are taking “root” in high-rise, science-fiction-inspired buildings on the fringes, or even within city limits.
Another example is meat substitutes that are grown, for the most part, in vats using microorganisms. And then there are the enthusiastic proponents of farmed insects high in protein. How about some curried cockroach for dinner?
Food in the future will no doubt reach our plates via a greater diversity of technology and systems. Some “next gen” foods might look, taste, and smell a lot like current-gen foods, but differ greatly in other ways. For some next-gen foods, the differences will be recognized, tested to some degree, and perhaps even adequately vetted with consumers. But others likely won’t be.
Here on Hygeia Analytics, we will try to tip the odds toward deeper knowledge and understanding of the full range of consequences buried in the genes of next-gen foods, before we are all consuming them for breakfast without even knowing it.
Lab-Grown Meat
Big money is flowing into fake-meat startups all over the world, in pursuit of three laudable goals —
- Lessening animal agriculture’s contribution to global climate change.
- Slowing the conversion of rainforests to cattle pasture and crop farms producing animal feed.
- Reducing the number of animals subjected to inhuman treatment on CAFO-scale operations.
One of the first hurdles for lab-grown meat in the U.S. will be regulatory. Will these products be regulated like meat, or does artificial food fall into a category all its own? But first, agencies have to play a bit of tug-of-war to decide who gets to regulate these new products – USDA which has jurisdiction over meats, while FDA is also maneuvering to be the lead agency. We will follow this closely as it develops.
Other Resources:
- ABC News Story – “The ‘beef’ on lab-grown meat: FDA hosts public meeting to make case for oversight,” July 2018
Towards Healthier Fats
Our understanding of what makes a fat healthy or unhealthy has shifted dramatically in the last decade. In a nutshell, we now know that the quality of fat in a person’s diet is as critical as quantity.
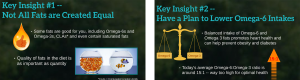
As more is learned about the importance of a healthy fat intake, agricultural companies are sitting up and taking notice. New research is looking into ways we can maximize our intake of healthy fats, while reducing intake of those that are pro-inflammatory and clearly not heart-healthy.
High-Oleic Soybeans
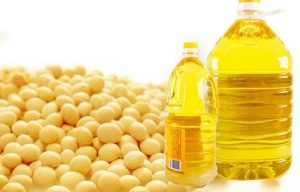
One example of a red-hot area of innovation is the development of cooking oils that do not contain now-banned industrial trans fats.
Soybean oil was widely used to produce partially-hydrogenated oils for use in processed foods. These oils were more stable in baked goods and they extended the life of oil needed to satisfy demand for French fries, fish and chips, fried chicken, and many other deep-fat fried foods.
But the partial-hydrogenation process that makes cooking oils more stable has the unintended consequence of also producing unnatural trans fats linked to heart disease, cancer and other health problems (which is why the FDA has banned them).
Soybean genetic engineers are working hard to produce high-oleic soybeans with reduced amounts of other fatty acids that tend to break down when heated, turning oil rancid. Oleic acid is a fatty acid that is relatively stable, even when heated, and extends the shelf life of baked and fried foods.
Soybean breeders at other seed companies are also working to develop high-oleic soybeans without using genetic engineering. Some of these companies also sell GE crops, but have concluded it will be cheaper for farmers, and better overall, to use conventional breeding to alter the fatty acid profile of soybeans in a way that makes partial hydrogenation unnecessary.
But as in all paths leading to the future of food, there are complications in this quest for stable cooking oils for baking and deep-fried foods. First and foremost, high-oleic soybeans have lower concentrations of heart-healthy omega-3 fatty acids that we all need more of (see our Primer on Fatty Acids for an in-depth look). Second, is cooking food in fat really the best option?
Producing Milk High in Healthy Fatty Acids
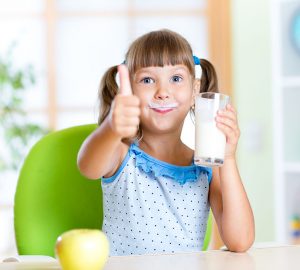
Parts of the dairy industry are also taking a hard look at healthy fats. American diets are too high in omega-6 fatty acids and way low in omega-3s. Increasing omega-3 intakes is essential in our national quest toward a healthier balance between these two types of fatty acids.
Dairy products are a big part of most American diets, and increasing the omega-3 fatty acids in milk could go a long way towards balancing fatty acid intake, as research featured on Hygeia Analytics has shown.
One study published in 2013 revealed that conventional dairy production markedly raises the omega-6 fatty acid concentration in milk, while lowering levels of omega-3s.
The reason why is clear as a bell — on conventional dairy farms, heavy feeding with corn and soybeans raises omega-6 levels in cow’s milk. A dairy cow needs forage-based feeds to provide the building blocks of omega-3 fatty acids.
Because organic farmers feed their cows much more forage-based feeds than their conventional dairy farm neighbors, organic milk averages around 62% more omega-3s than milk from conventionally managed cows — a very substantial difference with heart-health implications.
The omega-3s in milk come from the pasture and forage-based feeds in a cow’s diet, feeds that are relied on much more heavily on most organic dairy farms than on conventional dairies.
As the graphic below illustrates, the amount of forage in a cow’s diet varies greatly between management systems.
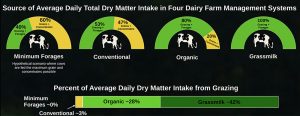
“Grassmilk” dairies produce milk from cows feed an essentially 100% forage-based, compared to around 50-50 forages and grains and “concentrates” on most conventional dairies.
In a nation-wide study featured on Hyegia, a team analyzed the impacts of nearly 100% forage-based feed on omega-6 and omega-3 levels in whole milk.
Grassmilk averaged 148% higher omega-3s than conventional milk, and 123% higher levels of conjugated linoleic acid, or CLA. CLA is another heart-healthy fatty acid that increases with forage feeding, especially from high-quality, fresh pasture.
Increasing the amount of forage-based vs. grain-based feeds in a dairy cow’s ration is just one example of how farmers are working to maximize the nutritional quality of human foods by enhancing the quality of fat. As long as consumers want and seek out healthier food choices, the quest on the farm, in the lab, and across the food industry will go on in the search for better ways to provide just that.
More Resources:
- Brad Heins, University of Minnesota, “Why Grass Matters”
- Charles Benbrook and Don Davis, “The Multiple Benefits of Grassmilk Dairy Production Deserve a Closer Look
- Melanie Jenkins, “New research claims health benefits of grass-fed milk,” Farmers Weekly – UK, March 1, 2018.
- Melody Meyer, “Got Milk from 100% Organic Grass-Fed Cows? It’s Healthier for You!,” Organic Matters, February 27, 2018
- 2013 PLOS ONE Paper on Conventional and Organic Milk
- Primer on Good Fat, Bad Fat